mycorrhizal fungi
Press coverage around SPUN and mycorrhizal fungal networks.
Nearly all plants on Earth form a symbiosis with mycorrhizal fungi. These fungi have altered the evolutionary history of the planet.
Mycorrhizal fungi are a group of network-forming soil fungi that form symbiotic associations with plants. Nearly all plants form symbiotic associations with mycorrhizal fungi. These associations have shaped life on earth for more than 475 million years.
Symbiotic relationships between plants and mycorrhizal fungi are around 475 million years old and play fundamental roles in the Earth’s biosphere.
Underground economics
Plants and fungi exchange resources with one another and are able to strike compromises, resolve trade-offs and deploy sophisticated trading strategies.
Soils are some of the most complex ecosystems on Earth, and fungi must trade to survive. Animals rely on a central nervous system to make trade decisions, but fungal networks must evaluate trade environments without a brain. First, they must forage for nutrients in the soil (like phosphorus and nitrogen). Second, they must exchange these nutrients for carbon compounds (like sugars and fats). To do this, mycorrhizal fungi have evolved sophisticated trading strategies, and can discriminate between plant partners, exchanging more resources to plants that provide them with more carbon. Fungi can capitalize on value differences across complex trade networks by moving resources to where they gain a better price from plant ‘buyers’. In one study, when faced with an unequal supply of nutrients across their networks, mycorrhizal fungi moved phosphorus to areas of scarcity, where it was in higher demand and therefore fetched a higher ‘price’. By doing so, the fungus was able to receive larger quantities of carbon in return. Fungi can even hoard resources until they retain a higher ‘price’. Researchers are using new tools to tag nutrients inside fungal networks and track fungal trade decisions.
SOURCES
Van't Padje A. et al. “Mycorrhizal fungi control phosphorus value in trade symbiosis with host roots when exposed to abrupt 'crashes' and 'booms' of resource availability”. New Phytol. 5 (2021) doi: 10.1111/nph.17055.
Noë R, Kiers ET. “Mycorrhizal Markets, Firms, and Co-ops”. Trends Ecol Evol. 3 (2018) doi: 10.1016/j.tree.2018.07.007.
Bogar LM. Et al. “Does resource exchange in ectomycorrhizal symbiosis vary with competitive context and nitrogen addition?” New Phytol. 3 2022 doi: 10.1111/nph.17871
Kiers et al. “Reciprocal rewards stabilize cooperation in the mycorrhizal symbiosis”. Science 12 (2011) doi: 10.1126/science.1208473.
Underground connections
Mycorrhizal fungi form networks that have the potential to connect plants underground. These networks may help distribute nutrients across ecosystems. Under laboratory conditions, organisms, like bacteria, can also use these fungal ‘super-highways’ for transport, allowing them to travel between between different roots.
Underground, mycorrhizal fungi form networks of hyphae potentially connecting roots of diverse host plants. The function of these networks, called common mycorrhizal networks (CMNs), has been debated for decades. In some cases, they may facilitate the flow of nutrients and carbon, mediate both cooperative and competitive relationships between plants, and help protect plants from pests and pathogens. Once a plant has ‘plugged into’ a CMN, the network may serve as a physical conduit for the movement of nutrients and chemicals. The vast majority of these studies have been done in the laboratory, so more field work is needed to understand the function of CMNs under natural conditions.
SOURCES
Beiler, K. J., et al. “Vertical partitioning between sister species of Rhizopogon fungi on mesic and xeric sites in an interior Douglas-fir forest”. Molecular Ecology 21 (2012)
Lian, C. et al. “Tricholoma matsutake in a natural Pinus densiflora forest: correspondence between above- and below-ground genets, association with multiple host trees and alteration of existing ectomycorrhizal communities”. New Phytologist 171 (2006)
Figueiredo, A. et al. “Common mycorrhizae network: A review of the theories and mechanisms behind underground interactions”. Frontiers in Fungal Biology 2 (2021)
Leake, J., et al. “Networks of power and influence: the role of mycorrhizal mycelium in controlling plant communities and agroecosystem functioning”. Canadian Journal of Botany 82 1016-1045 (2004).
Newman, E. “Mycorrhizal links between plants - their functioning and ecological significance”. Advances in Ecological Research 18, 243-270 (1988).
Robinson, D. & Fitter, A. “The magnitude and control of carbon transfer between plants linked by a common mycorrhizal network”. Journal of Experimental Botany 50, 9-13 (1999).
Selosse, M.A. et al. “Mycorrhizal networks: des liaisons dangereuses?” Trends in Ecology & Evolution 21 (2006)

Underground communication
Some researchers have published work to suggest that plants can pick up cues from neighboring plants via shared mycorrhizal networks, potentially allowing them to prepare for insect attacks. But much more evidence is needed before we understand if this is actual “communication.”
Babikova, Z. et al. “Underground signals carried through common mycelial networks warn neighbouring plants of aphid attack”. Ecology Letters 16: 835–843 (2013)
SOURCE
Babikova, Z. et al. “Underground signals carried through common mycelial networks warn neighbouring plants of aphid attack”. Ecology Letters 16: 835–843 (2013)
Underground flows
Phosphorus, nitrogen, carbon, and other nutrients flow through mycorrhizal networks in complex patterns. To understand how fungi coordinate flows, researchers are simultaneously monitoring the architecture of networks and the direction and speed of flows within the networks.
How do fungi control the flows of nutrients across their large and complex networks? Fungal networks are bathed in a rich field of sensory information and must integrate a complex array of chemical, physical, and environmental stimuli. Fungal networks must constantly remodel themselves, sending carbon to growing tips to build new trade routes, and collect nutrients, like phosphorus and nitrogen, to send to plants roots. Scientists are studying the complex flow patterns inside fungal networks to find out how we can better harness the power of mycorrhizal associations to store carbon and support the health of ecosystems.
SOURCES
Whiteside MD. et al. "Mycorrhizal Fungi Respond to Resource Inequality by Moving Phosphorus from Rich to Poor Patches across Networks". Curr Biol.(12) 2019 doi: 10.1016/j.cub.2019.04.061
Bago B. et al. "Translocation and utilization of fungal storage lipid in the arbuscular mycorrhizal symbiosis". Plant Physiol. (1) 2002.
THE FOUR TYPES OF MYCORRHIZAL FUNGI
There are four main types of mycorrhizal association. Each type interacts with plants in different ways and differ in their ability to store carbon and forage for nutrients.
1

ARBUSCULAR MYCORRHIZAL FUNGI
Arbuscular mycorrhizal fungi are the ancient, ancestral form of mycorrhizal symbiosis. These fungi played a key part in the movement of plants’ ancestors onto dry land. By the time the first roots evolved, the mycorrhizal association was already some 50 million years old.
Plants that associate with arbuscular mycorrhizal fungi make up around 70% of global plant biomass
Physiologically, arbuscular mycorrhizal fungi form intracellular structures in roots called arbuscules. Arbuscule means ‘a branched treelike organ’. This is because arbuscules look like mini-trees inside the roots of plants. Arbuscules are the main sites of nutrient exchange between plants and fungi. Plants associated with arbuscular mycorrhizal fungi represent around 70% of global plant biomass, including all major crops, making it one of the most important symbiotic relationships on Earth.
2
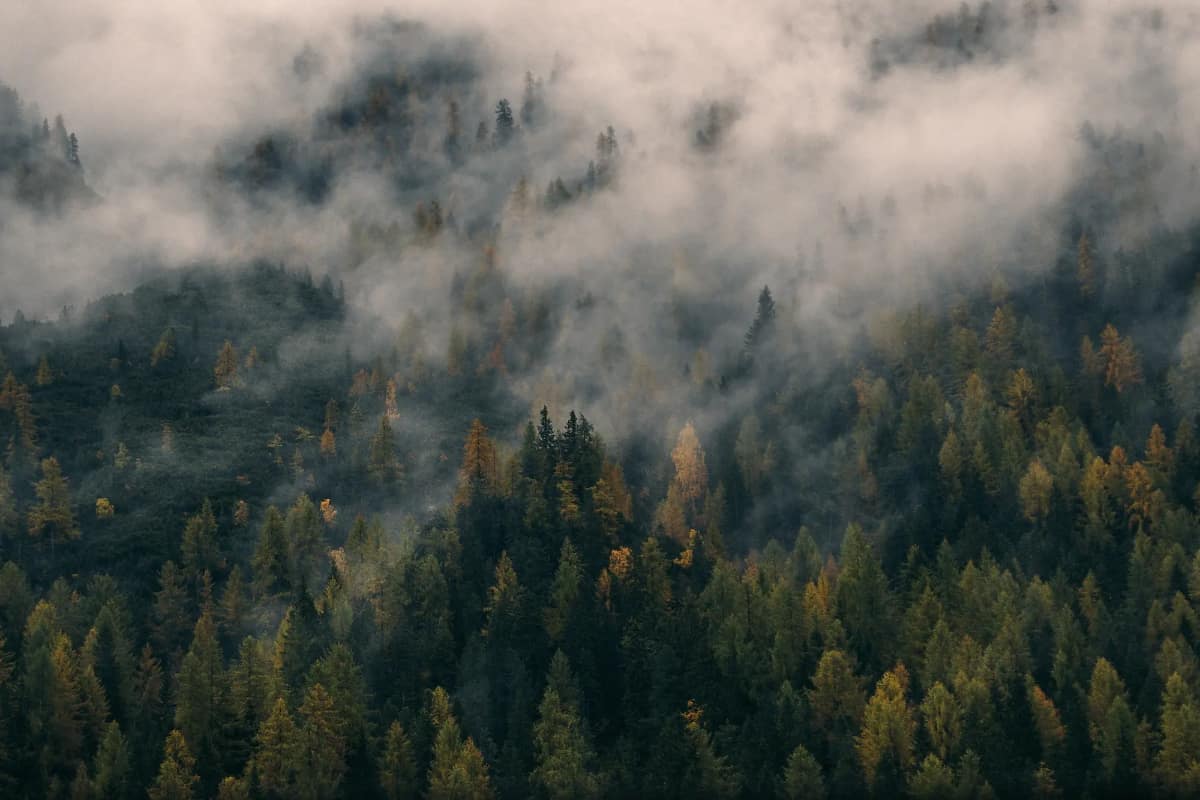
ECTOMYCORRHIZAL FUNGI
Trees in most boreal and temperate forests depend on ectomycorrhizal associations. Ectomycorrhizal associations have evolved more than seventy times since the first movement of plants onto land. These fungi form a mycelial sleeve around plant root tips, called a ‘Hartig net’, where exchange of nutrients and carbon takes place. Unlike arbuscular mycorrhizal fungi, ectomycorrhizal fungi do not grow into plant cells (‘ecto’ means outside).
The ectomycorrhizal lifestyle has evolved independently more than 70 different times
Ectomycorrhizal fungi evolved from free-living decomposer fungi and retain a wide range of enzymes that allow them to degrade complex substances in soils. Ectomycorrhizal fungi are excellent foragers and are able to obtain nutrients unavailable to arbuscular mycorrhizal fungi. They require more energy from their plant partners than arbuscular mycorrhizal fungi, and tend to form relationships with shrubs and trees.
3

ORCHID MYCORRHIZAL FUNGI
Most plants supply their mycorrhizal partners with carbon in exchange for mineral nutrients. Orchids are able to do something different, and obtain both carbon and mineral nutrients from their fungal partners for at least some of their lives.
~17,000 species of orchids rely on very specialized fungal partnerships for nutrients
Of all the plant families, orchids are the most diverse, and orchid-specific mycorrhizal fungi may have played a role in their evolutionary success. There are 250 species of orchid that have lost the ability to photosynthesize entirely, and acquire all the carbon and nutrients they need to survive from their fungal partners.
4
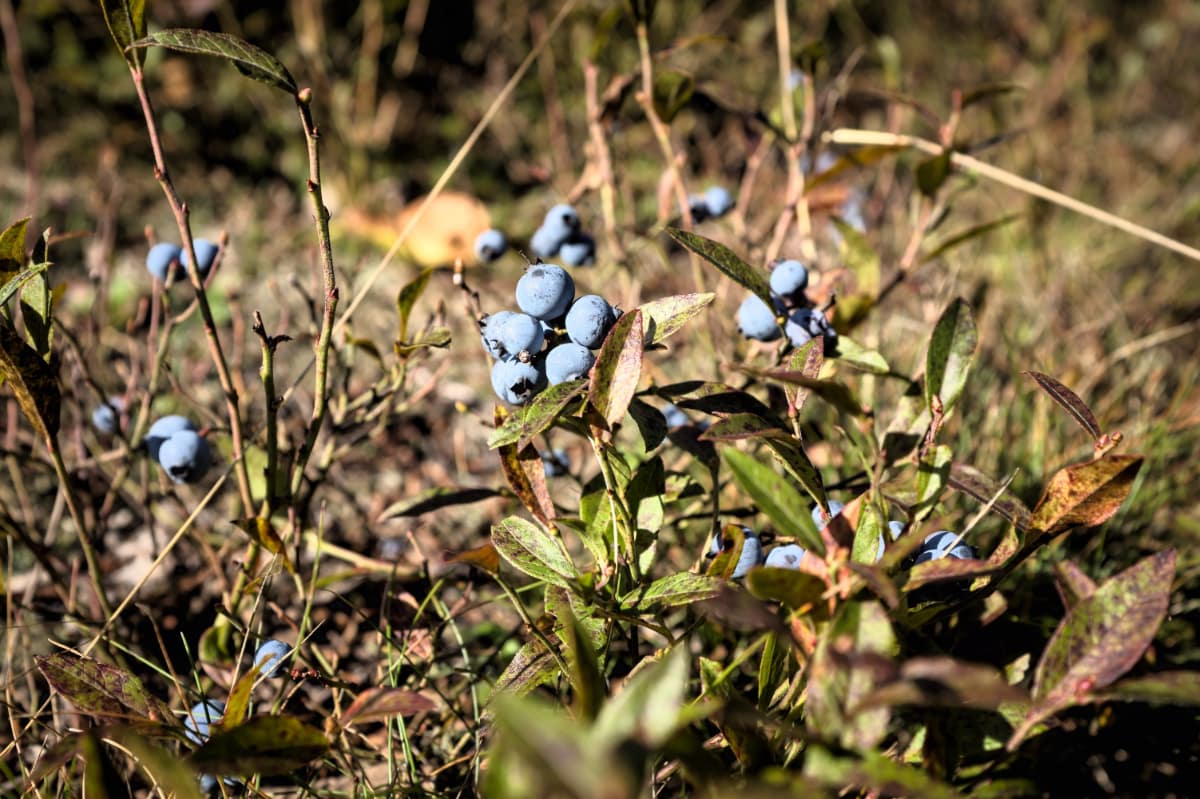
ERICOID MYCORRHIZAL FUNGI
Ericoid mycorrhizal fungi form relationships with plants in the family Ericaceae, which includes heather, blueberries and cranberries. They are most commonly found in acidic and infertile soils, including bogs, heathlands, and boreal forests.
Ericoid mycorrhizal fungi produce enzymes which allow them to break down complex organic molecules
Ericoid mycorrhizal fungi form coils in the root cells of their plant partners rather than arbuscules, and produce enzymes that allow them to break down complex organic molecules.